RESEARCH
BLIND PLANKTON MANAGES TO SWIM... HOW?!
Copepods make up the majority of the plankton found in the sea: the survival strategies of these species is a great evolutionary success! But how do these (almost) blind micro-organisms detect and distinguish preys to feed, other copepods for reproduction or predators to escape, while swimming in a turbulent environnement?
Copepods have highly sensitive antennae, which they use to mesure hydrodynamical signals. I developed a protocol and installed experiments during my postdoc at IRPHE, in the group of Christophe Eloy, to investigate how copepods react to a typical fluid stimulation. Constructing a mini lab took a bit of time, and growing happy phytoplankton is a necessary challenge to feed the copepods. I supervised a master student and we succeeded in growing the phytoplankton and even observed adult copepods! This exciting news validated my protocols, and we stay in touch for the next steps.
Before we share our observations about detection and swimming, here is a gallery of what copepods look like, that I found on the twitter account @copepodology:
LIGHT PRODUCTION IN DINOFLAGELLATES

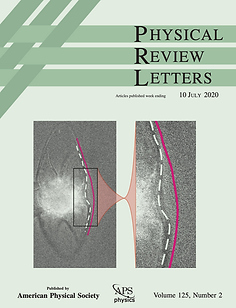
Dinoflagellates are often responsible of algal blooms and can light up the sea at night (the "disco-plankton"!).
We explored the mechanisms triggering the emission of light in the dinoflagellate P. Lunula. The internal chemistry leading to light is rather well known (it involves luciferin and luciferase), but how an outside mechanical stimulus triggers it is by far more mysterious! I took part in the study that shoots a jet of liquid to the Lunula, to mimic the flow created by a nearby swimming predator, and colleagues continued with mechanical stimulation. All of this work is explained there, and here, and there...
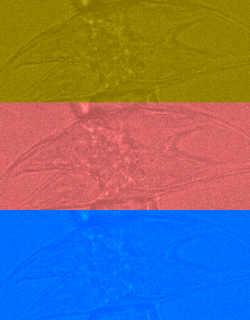
PHOTOTACTIC SWIMMING OF MICRO-ALGAE
I studied the swimming of a fascinating microscopic green algae, Gonium. This flat tiny colony of 16 cells is at the edge of multicellularity, and can teach us a lot about the emergence of locomotion, and coordinated motion in simple organisms. Indeed, this algae manages to swim efficiently towards light: how does that happen?
Explanations here!
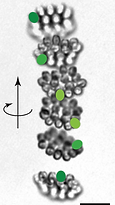
AEROPHILICITY
A superhydrophobic surface repels water as it is preferentially covered by air. If such a surface is immersed in a pool, it will still repel water and stay preferentially covered by air. In this context, it can therefore be described as aerophilic!
Spreading of bubbles
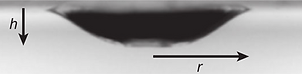

The dual experiment of the spreading of a drop on a wetting surface is the spreading of an air bubble on an aerophilic surface! We describe here the dynamics of this reversed situation, with unusual laws as the main viscous dissipation does not happen in the bubble (as it would for a drop) but in the surrounding liquid.
Tightrope bubbles
The dual situation of a drop rolling down a fibre is a bubble sliding upwards on an aerophilic fibre! The bubbles, once captured by the fibre are guided in a controlled way to the surface. We describe here the friction law that limits the bubble velocity.

Capillary descent
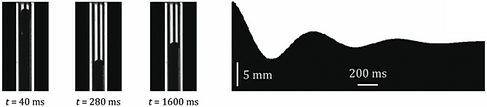
The dual experiment to the capillary rise is the descent! To achieve this, with Joachim Delannoy we used aerophilic capillary tubes, immersed in a bath and initially filled with water. We describe here the dynamic of the descent of the meniscus once the tube is brought in contact to the water/air surface.
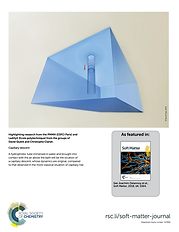
CRENELATED FRICTIONS
Water droplets show an exceptional mobility on superhydrophobic surfaces, running at high speed in any direction. In these works we try to control both direction and velocity, by introducing obstacles. Playing with the shape of the texture and the droplet viscosity enables to describe and characterise various regimes of frictions. These experiments show a wide variety of drop shapes: See here for a complete explanation of the friction on symmetrical ridges and here for the unexpected behaviour of droplets on wider ridges.
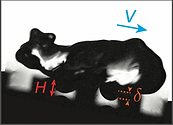

AIR-PROPELLED PLATELETS
A platelet above an air-table levitates. A texture redirects the air-flow. An asymmetry in the texture creates a preferred direction for the air-flow. This enables propulsion of the levitating platelet.
With Dan Soto, we designed two symmetrical situations: first, the texture is engraved in the air-table, and the flowing air will drag by viscosity the levitating plate, as explained here. We also engraved the texture on the levitating plate, and in this configuration inertial effects will be dominant, leading to a propulsion in the other direction! This is also detailed here.

DROPLET IMPACTS
When a drop falls on a liquid bath it captures a small air bubble. Investigating this phenomenon, we noticed that the bubble volume is twice the volume of a bubble captured in the case of a drop impact on a solid surface, or of a solid sphere impact on a liquid bath: indeed in our experiments, there are two deformable surfaces, the drop and the bath! All this is explained here.
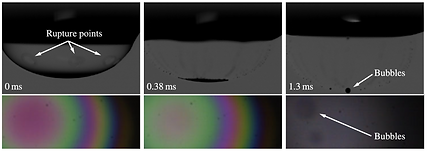
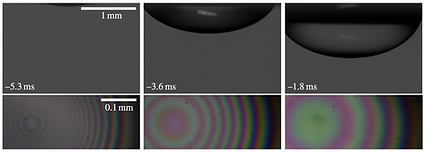